In this blog we are going to share the basic challenges and treatment of myocardial infarction symptoms. Coronary atherosclerotic cardiovascular disease and acute myocardial infarction (MI) are still some of the major contributors to high rates of morbidity, mortality, disability, and limited treatment options available.
For this reason, these two circumstances have always been important to cardiologists, leading to significant research and improved treatment outcomes. Although pharmaceutical and therapeutic approaches for myocardial ischemia-reperfusion are continually improving, the annual incidence rate of heart failure is rising.
Researchers believe therapies that do not directly target the infarcted myocardium cause this situation. Some examples include medications, rehabilitation, or reperfusion therapy after an ischemic injury. These approaches offer help but do not fully address the problem.
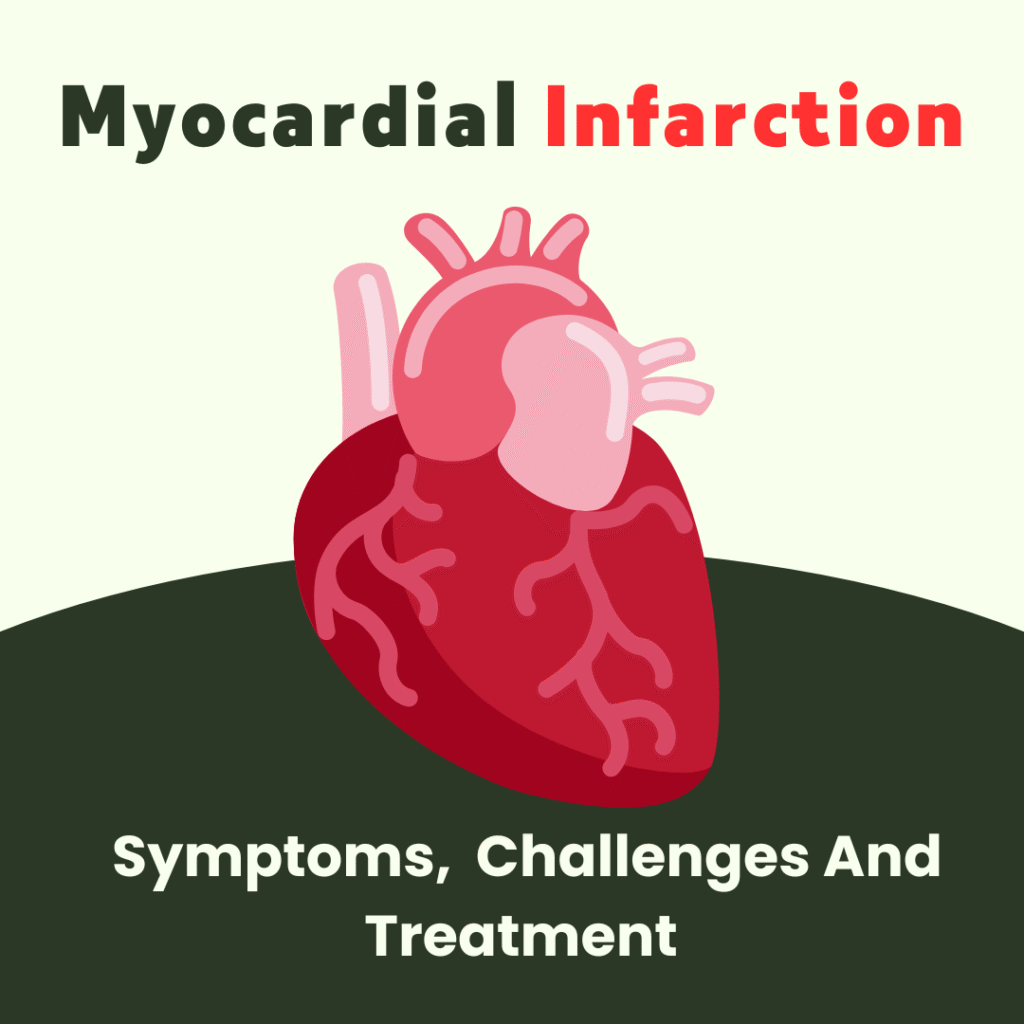
Therefore, there is a persistent and growing case of heart failure following myocardial infarction, as there is no intervention targeting root causes such as myocardial disturbance or damaging mechanisms that cause a decline in cardiac function over time. This involves examining the stages of therapy but also highlights the need for new methodologies in treating patients with this disease.
Nanoparticles may present themselves as viable prospects because they possess together remedial and preventive properties for acute myocardial infarction (MI). These new multidimensional nanoparticles may be more effective against the disease. Although many advances have led to decreased mortality from acute myocardial infarction, there is still a lot to be done in reducing heart failure rates.
As a result, current studies on these Nano-sized particles mark a move towards better management of myocardial dysfunctions accompanied by their associated complications.
Introduction:
Cardiovascular disease is the number one cause of death globally, with approximately 31% of all global deaths falling into this category. Out of these, myocardial infarction (MI) is particularly deadly and it causes 13% of heart-related deaths. The closure of the coronary artery causes MI (myocardial infarction), hence preventing blood from flowing to the heart either partially or completely.
This pause in the flow of blood leads to the death of the surrounding myocardium. Since the heart has a limited capacity to regenerate cardiomyocytes endogenously, eventually fibrous cells replace the lost myocardium cells. This process of replacement leads to the poor contractile ability of the heart, often resulting in end-stage heart failure.
Standard treatments for MI include thrombolytic therapy, immediate percutaneous coronary intervention (PCI), and administration of lipid-lowering medications and anticoagulants. These approaches have proven effective in reducing infarction size and mortality rates. For example, percutaneous coronary intervention can restore blood flow through the affected coronary artery.
However, this procedure carries the risk of ischemia-reperfusion injury, leading to additional tissue damage and myocardial cell apoptosis. In the end, these complications may contribute to heart failure and other negative health outcomes.
Post-MI pathological changes within the body, such as the production of reactive oxygen species, mitochondrial dysfunction, and calcium overload, worsen the problems. These changes cause cell death and inflammatory responses that hinder the recovery process.
Despite the existence of anti-inflammatory and antioxidant medication, their efficacy is usually reduced by limited specificity, low bioavailability, and short half-life. Hence, there is an urgent need for safer and more economical ways to prevent and manage myocardial infarction.
Current Practices:
Extensive research and discussions over the past decade have informed current therapeutic practices on myocardial infarction. For instance, healthcare providers should administer thrombolytic medications at the earliest possible time for maximal benefit.
However, thrombolysis may only be fully effective if administered up to 12 hours after symptoms appear. Healthcare providers can use audit reviews to help ensure timely administration by monitoring and improving the timing of thrombolysis.
Additionally, healthcare providers must perform electrocardiograms (ECG) within 15 minutes of the patient’s arrival at the hospital and commence thrombolytic therapy within 1\2 hours. The GUSTO-I trial assessed the use of tissue plasminogen activator (TPA) and streptokinase for occluded arteries around the world and found that TPA is better than streptokinase in some patients.
This includes younger individuals, those who come in earlier, and people who had anterior infarcts or myocardial infarction before being treated with streptokinase. Although researchers have not defined the mechanism of action for aspirin, it seems to be as effective as thrombolytic drugs and is considered a life prescription post-MI.
Furthermore, evidence suggests that early treatment with angiotensin-converting enzyme (ACE) inhibitors and β-blockers can enhance long-term prognosis in patients with decreased ventricular function. However, significant evidence does not support the use of prophylactic antiarrhythmic drugs or nitrates.
Recent Treatments:
1) The Development of Regenerative Heart Medicine:
Regenerative biology examines how organisms create new tissue to replace or repair damaged and lost tissues. By the next generation, human genetics, stem cell technology, and reprogramming techniques will transform cardiovascular biology as we know it today. Now we can say that regenerative medicine is moving from being a concept to a reality; practical applications are coming forth into focus.
Researchers classify human cells according to their regeneration capabilities. They have long thought that striated muscle cells like cardiomyocytes contributed minimally to healing after injury and had poor regenerative abilities; these kinds of cells have often healed by scarring plastic.
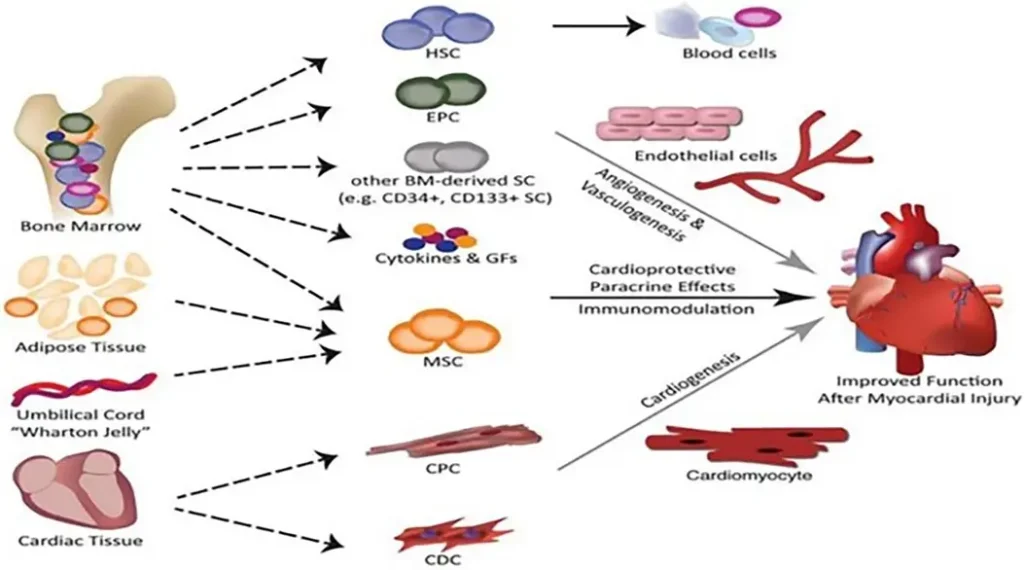
However, almost two decades of heart regenerative medicine research have disproved this view. Researchers in this century have discovered that tiny portions of myocyte nuclei beyond the infarct area show cell division markers indicating there might be some regenerative potential in cardiomyocytes.
Nevertheless, the quantity of cardiomyocyte self-regeneration stays below one percent per year, making it unlikely to suggestively influence remedial ensuing myocardial infarction.
2) Cells in Myocardial Regeneration:
Researchers should obtain the ideal cells for cardiac tissue regeneration and repair from the patient, with strong control mechanisms, localized activity, and the potential to differentiate into various cardiovascular lineages. Once implanted in the damaged myocardium, these cells are expected to promote the long-term regeneration of the tissue.
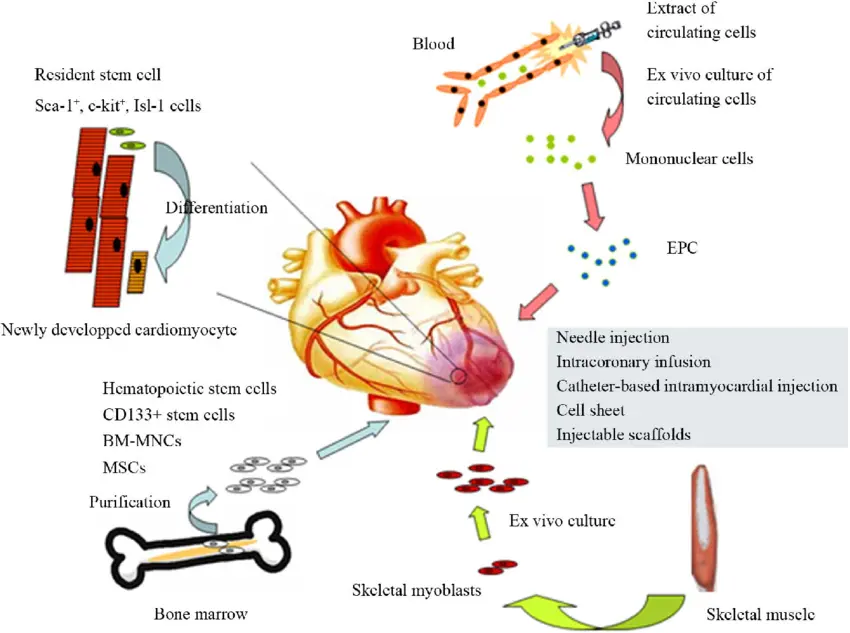
Several stem cell types have been explored for their potential in myocardial repair, including hematopoietic stem cells (HSCs), embryonic stem cells (ESCs), myeloid stem cells (MSCs), induced pluripotent stem cells (IPSCs), skeletal myoblasts, and endothelial progenitor cells.
3) Stem Cell Therapies for Cardiac Repair:
1) Mesenchymal Stem Cells:
Mesenchymal stem cells (MSCs) belong to a group of enthusiast pluripotent cells that can differentiate into various specific cell types such as adipocytes, osteoblasts, and chondrocytes. Researchers can isolate them from bone marrow, fat, or other sources. MSCs express several cytokines including stem cell factor (SCF) and interleukin 1& 6 (IL1& IL6).
In normal physiological conditions, however, MSCs have inadequate ability to transform into functional cardiomyocytes. Instead, they play an important role in cardiac repair through paracrine signaling rather than differentiation into actual heart muscles.
MSCs release factors like fibroblast growth factor (FGF) and vascular endothelial growth factor (VEGF), promoting angiogenesis and reducing tissue ischemia, ultimately supporting cardiac perfusion.
2) Induced Pluripotent Stem Cells:
Induced pluripotent stem cells (IPSCs) are promising candidates in cardiac repair. Researchers found that an infusion of a mix of cardiomyocytes, smooth muscle cells, and cells derived from IPSCs into a pig model of acute myocardial infarction restored the functioning of the heart without causing any ventricular arrhythmias.
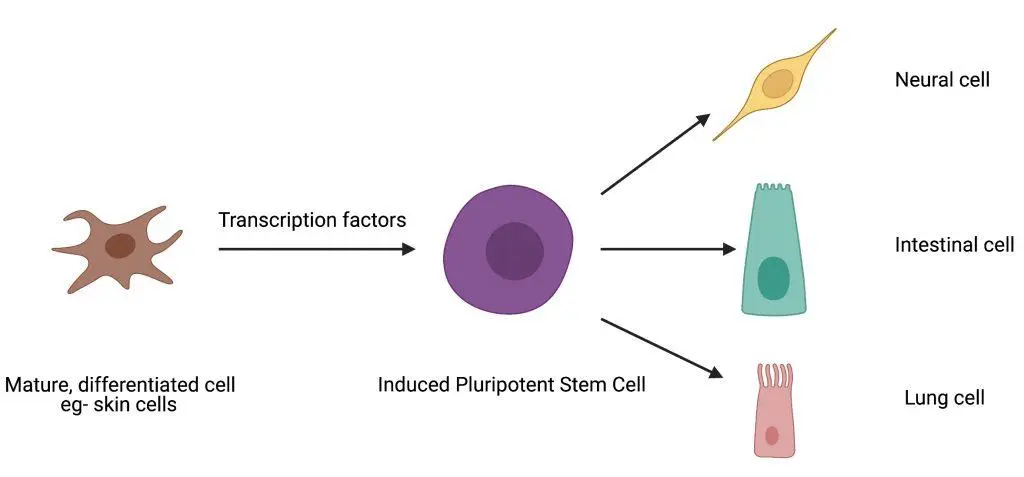
The study proved that it is safe to use IPSC treatment to restore the heart. Describing pluripotency induction in differentiated cells, transcription factors regulate the procedure of converting a mature cell into an induced pluripotent stem cell, which can then be directed to differentiate into any desired cell type.
In addition, implanting a large patch made up of these mixed cells into the pig model led to remarkable improvements in cardiac performance and left ventricular remodeling.
3) Cardiac Progenitor Cells:
Cardiac progenitor cells (CPCs) derived from the heart’s tissue can develop into cardiomyocytes or heart muscle cells. Recent studies demonstrated the potential regeneration of myocardial cells by some CPCs but noted that their differentiation rates are low, hindering their therapeutic applications on human beings.
Various preclinical trials using growth factors, microRNAs, and other agents show that there is hope for enhancing the differentiation capacities of CPCs, possibly culminating in clinical therapies someday.
In one small pig model with myocardial infarction, transplantations using CPCs enhanced cardiac functions and reduced the size of the myocardial scarred area.
Researchers need to conduct clinical studies to evaluate safety as well as their possible effects on human beings after preclinical findings suggest so.
4) Use of Nanotechnology:
Nanoparticles (NPs), usually measuring from 1 to 100 nm in diameter, have found extensive applications in medicine due to their diverse shapes, surface chemistries, and structures. Nanotechnology-based drug delivery systems offer more advantages than conventional methods such as lower costs, increased bioavailability, and enhanced drug efficacy among others.
Researchers use these systems to prolong drug administration time, modify routes for administration, and reduce therapeutic dosages. Common carriers for drug delivery include polymer nanoparticles, dendrimers, micelles, Nano gels, liposomes, and solid lipid nanoparticles.
They are being researched for their potential in the treatment and prevention of myocardial infarction (MI), whereby the heart muscle becomes damaged due to low blood supply. Researchers also previously exploited these particles to treat tumors in addition to neurological disorders, while research is ongoing concerning their applicability in the management of cardiovascular diseases.
They help deliver medicinal agents directly into specific locations within the body with great precision, resulting in minimal side effects and improving therapeutic outcomes significantly.
- For example, NPs can deliver antioxidants to infarcted regions of the heart, reducing oxidative stress and promoting healing.
Studies have shown that NPs loaded with specific peptides can target ischemic myocardium and mitochondria, offering cardioprotective benefits. Additionally, NPs like puerarin-SLNs have demonstrated a reduction in infarction size in animal models of MI.
Biocompatibility of Nanoparticles:
Ensuring NPs’ biocompatibility is crucial for their therapeutic application. The interaction between NPs and cells or molecules can result in potential toxic effects; hence, predicting NP biocompatibility is difficult. NPs usually move around the body through the blood, but they can also accumulate in organs such as the kidneys and liver, leading to toxicity.
Factors leading to NP toxicity include oxidative stress, free radical production, and particle material composition. Researchers use functionalization with non-toxic surface molecules targeting delivery and stabilization as strategies to reduce NP-associated toxicity.
These methods have been shown to reduce adverse effects and enhance therapeutic efficacy. Nanotechnology-based drug delivery systems are equally significant. They may improve medication stability, minimize side effects, and provide controlled release of drugs among other functions.
- For instance, NP systems that deliver plant-based medicinal compounds, usually used in traditional Chinese medicine, are being explored.
New approaches include using antioxidant delivery nanoparticles that target oxidative stress during MI treatment through infarcted myocardium therapy.
These materials, made from bioactive hydrogels or Nanofibers, possess mechanical elasticity, electrical conductivity, and the ability to encourage heart tissue repair. For instance, Nano gels, which are 3D structures made from cross-linked biopolymer networks, have been shown to improve heart function and reduce inflammation in animal models of MI.
4) Injectable Hydrogel Materials for Treatment of Myocardial Infarction
1) Designing Injectable Hydrogels:
Injectable hydrogels have gained significant attention in the fields of tissue engineering and drug delivery due to their unique properties. These hydrogels are composed of hydrophilic polymers that can retain a substantial amount of water, making them highly biocompatible and conducive to supporting cellular processes.
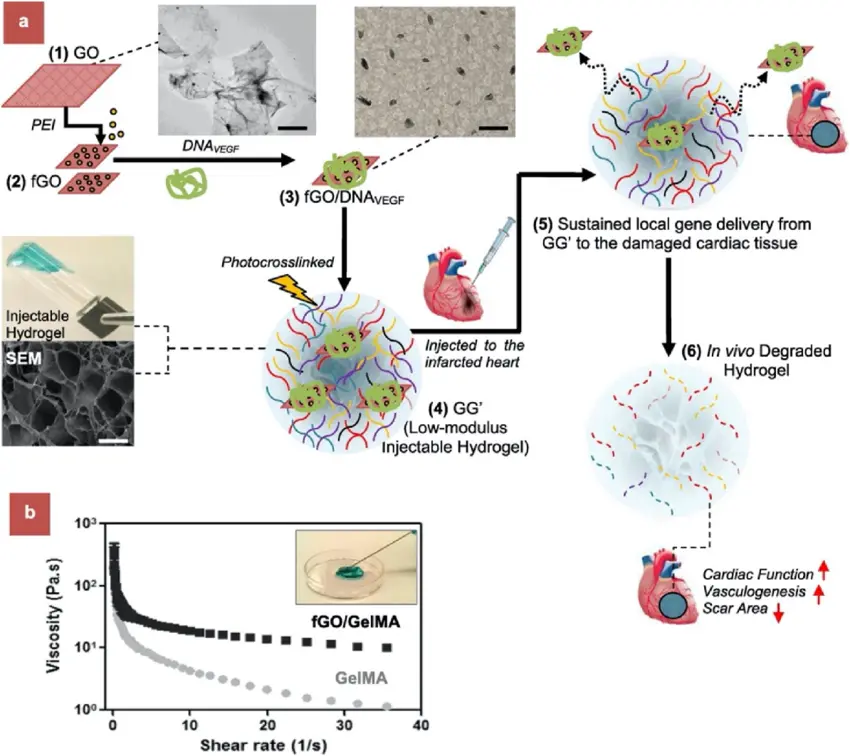
In the context of myocardial infarction (MI), injectable hydrogels offer a promising therapeutic approach. When injected into the myocardium, these hydrogels undergo a sol-gel transition in response to physiological conditions, enabling solidification in situ and providing structural support for damaged heart tissues.
Because of the adverse remodeling that happens in the heart’s left ventricle after MI, which can result in an expanded ventricle and drops in cardiac function, this support is really important. Injecting hydrogels that can condense the ventricular wall will change the geometry of the left ventricle, thereby reducing strain on the heart and enhancing its recovery.
A study found that changing the degree of methacrylic acid substitution in hyaluronic acid methacrylate polymers could improve hydrogel mechanical characteristics, enabling them to support the heart more effectively post-MI.
2) Preparation of Injectable Hydrogel for Acute Myocardial Infarction (AMI) Therapy:
These hydrogels have a natural extracellular matrix-like structure of the myocardium, offering a great advantage. Such a biomimetic structure helps maintain the viability of myo-cards stroma cells when injected into cardiac tissues either in vivo or ex vivo. This cell conservation is crucial for encouraging tissue regeneration and repair.
- For example, it has been shown that smooth muscle cells and endothelial cells move inside hydrogels, contributing toward recovering damaged heart muscles.
In other studies, injecting late-stage infarcted muscle with biocompatible synthetic recombinant human collagen type I (RHCI) matrices has restored cardiac mechanical properties and reduced scar formation.
This approach helps maintain cardiac function by preserving the thickness of distal walls and preventing further heart expansion.
These hydrogel-based therapies represent a bionic platform offering a safe and effective method for endogenous tissue repair, giving hope in treating myocardial infarction. Intramyocardial chitosan-based hydrogel injections have been shown experimentally to control infarction size, maintain ventricular wall thickness, and improve heart function globally.
Chitosan hydrogel stands out because it not only provides mechanical support but also creates a more favorable microenvironment for the myocardial repair process. These hydrogels may facilitate stem cell implantation, survival, and homing toward the ischemic heart.
Moreover, these hydrogels can scavenge reactive oxygen species (ROS) and chemokine, thus enhancing myocardial recovery, and making them versatile components of cardiac treatment options.
3) Major Challenges in Treating MI:
One of the major challenges in treating myocardial infarction (MI) is dealing with scar tissue that can lead to ventricular dysfunction. Scar tissue disrupts the myocardial conductance pathway, preventing the effective transmission of electrical impulses and slowing down local contraction. Researchers have created conductive biomaterials by introducing polypyrrole (PPY) into chitosan models to solve this problem.
These conductive hydrogels can be injected intramuscularly to restore synchronous ventricular contraction and allow the spread of electrical impulses through scar tissue. In summary, injectable hydrogels play several key functions in the treatment of myocardial infarction:
1) To Decrease Oxidative Stress:
Oxidative stress is a critical factor for understanding myocardial infarctions. Reactive oxygen species (ROS) are highly reactive molecules produced as byproducts of aerobic metabolism. Normally, ROS serves as signaling molecules and regulates diverse cellular processes, including contractile protein modulation and calcium handling.
However, after an MI, ROS production increases significantly, leading to oxidative stress. This excess production of ROS can damage cardiac cells, resulting in necrosis or apoptosis, contributing to adverse cardiac remodeling and contractile dysfunction.
Nicotinamide adenine dinucleotide phosphate (NADPH) oxidase, specifically the NOX2 isoform, mediates oxidative stress. NOX2 gets upregulated upon MI incidence, and its activation results in the increased generation of these molecules, causing more harm to the myocardium.
Researchers have made temperature-responsive hydrogels made up of N-isopropyl acrylamide (NIPAAM) and polyethylene glycol methyl methacrylate (MPEGMA) to address this issue. These hydrogels have ROS-scavenging abilities, reducing oxidative damage, increasing wall thickness, and consequently improving heart function in animal models of MI.
The ability of these hydrogels to search for ROS is crucial for protecting the myocardium in vivo. By lowering ROS levels, these hydrogels can prevent apoptosis, decrease membrane lipid peroxidation, reduce inflammation, maintain cardiac function, and promote angiogenesis in the left ventricle.
This complex approach to reducing oxidative stress can significantly improve outcomes following myocardial infarction.
2) To Encourage Angiogenesis in the Infarct Region:
Ischemic tissues are healed by a critical process known as angiogenesis, or the formation of new blood vessels. Following an MI, the heart struggles to get enough nutrients and oxygen needed for repair, which can deteriorate other functions of the heart. Delivering pro-antigenic signals to the infarcted area can inspire angiogenesis.
One of the most powerful pro-angiogenic factors is vascular endothelial growth factor (VEGF). Researchers have developed a peptide-based hydrogel that mimics VEGF. A peptide that mimics VEGF on its surface activates VEGF receptors on endothelial cells, leading to increased angiogenesis and enhanced biological activity in the injured myocardium.
Additionally, other studies have used citrate-based hydrogels, which are formed by combining citric acid with polyethylene glycol diol.
When injected into the left ventricle of rats with myocardial infarction, these hydrogels encourage angiogenesis and cardioprotection. The by-products of citrate metabolism activate the PI3K-Akt-mTOR signaling pathway and influence the citric acid cycle, both essential for promoting the development of new blood vessels and protecting the heart from further damage.
3) To Prevent Metalloproteinase-Induced Malignant Remodeling:
A heart attack initiates molecular-level changes in the heart, leading to the activation of an enzyme called MMP, which breaks down the extracellular matrix. An imbalance between MMPs and their tissue inhibitors, TIMPs, can lead to adverse ventricular remodeling.
Ventricular walls thin out, causing heart failure over time due to this remodeling process. Researchers have used hydrogels to deliver TIMPs into post-infarcted areas to counter these effects. Hyaluronic acid-based hydrogels deliver recombinant tissue inhibitors of metalloproteinases-3 (rTIMP-3) to the infarct region.
The local administration of TIMP-3 stimulates myofibroblast proliferation, preventing malignant remodeling after myocardial infarction (MI). This is essential for maintaining the heart’s structural integrity and improving its long-term outcomes.
4) To Increase Myocardial Electrical Conductivity:
The heart’s effective function depends on the synchronization of cardiac contractions. After an MI, the loss of viable cardiomyocytes and deposition of scar tissue can lead to arrhythmias and decreased cardiac output by disrupting electrical conductivity. Researchers have created conductive hydrogels to restore electrical connectivity in damaged myocardium.
- For example, hydrogels made using oligo(poly(ethylene glycol) fumarate) (OPF) integrated with graphene oxide (GO) nanoparticles not only offer mechanical support to the infarcted area
But also electrically couple isolated cardiomyocytes with adjacent healthy tissues. This helps return to synchronized contractions, improving overall heart function. Studies indicate that these hydrogels can stimulate increased expression levels of gap junction proteins like connexin 43 (Cx43), which is vital for cell-cell communication within the heart.
Researchers have also discovered the use of double-network hydrogels containing two interconnected networks: a flexible, hydrophilic, biocompatible, and cross-linked methacrylate aminated gelatin (MAAG) network, and a stiff, hydrophobic, and conductive poly (thiophene-3-acetic acid) (PTAA) network.
By adjusting the proportion between these networks, it is possible to fine-tune the hydrogels’ inflammation, and mechanical, and electrical properties, making them versatile tools for cardiac therapy.
5) Combination Treatment:
Myocardial infarction therapy is complex and necessitates addressing several issues such as extensive myocardial cell death, negative micro-environmental changes, loss of electrical conductivity in fibrotic scars, and insufficient blood supply to the affected area.
Combinatorial therapies based on multiple treatment strategies are emerging. One method involves integrating endothelial nitric oxide synthase (ENOS)-lipid/plasmid Nano complexes in soft conductive gels. These gels mimic the anti-fatigue and conductive properties of natural heart tissue, significantly increasing nitric oxide (NO) levels, which are essential for proper vessel and heart function.
When co-encapsulated with adipose-derived stem cells (ADSCs), these hydrogels promote the expression of angiogenic and cardiac-specific genes such as VEGFA, Ang-1, Cx43, and Cdh-2, thereby boosting heart function, increasing angiogenesis, and limiting fibrosis.
Conclusion
To summarize, injectable hydrogels are innovative materials for treating myocardial infarction. They provide a mechanical buffer to resist stress, facilitate blood vessel growth, inhibit negative remodeling, and restore electrical conductivity in injured tissues. As research progresses, future instructions on influencing stem cells involved in this therapy will likely rely on non-viral gene delivery strategies based on nanoparticles.
These treatments have a wide array of applications and may be crucial in combating many cardiovascular diseases that cause significant mortality rates globally. Detailed studies are necessary because we don’t yet fully understand how these hydrogels promote healing, including their ideal volume for injection, duration, agent concentration, and hydrogel degradation rate.
Increasing knowledge about these parameters suggests that hydrogel-based therapies will soon become common for myocardial infarction therapy, helping patients living with this illness.